Sessile marine animal Bugula neritina a highly studied marine invertebrate for medicinal uses
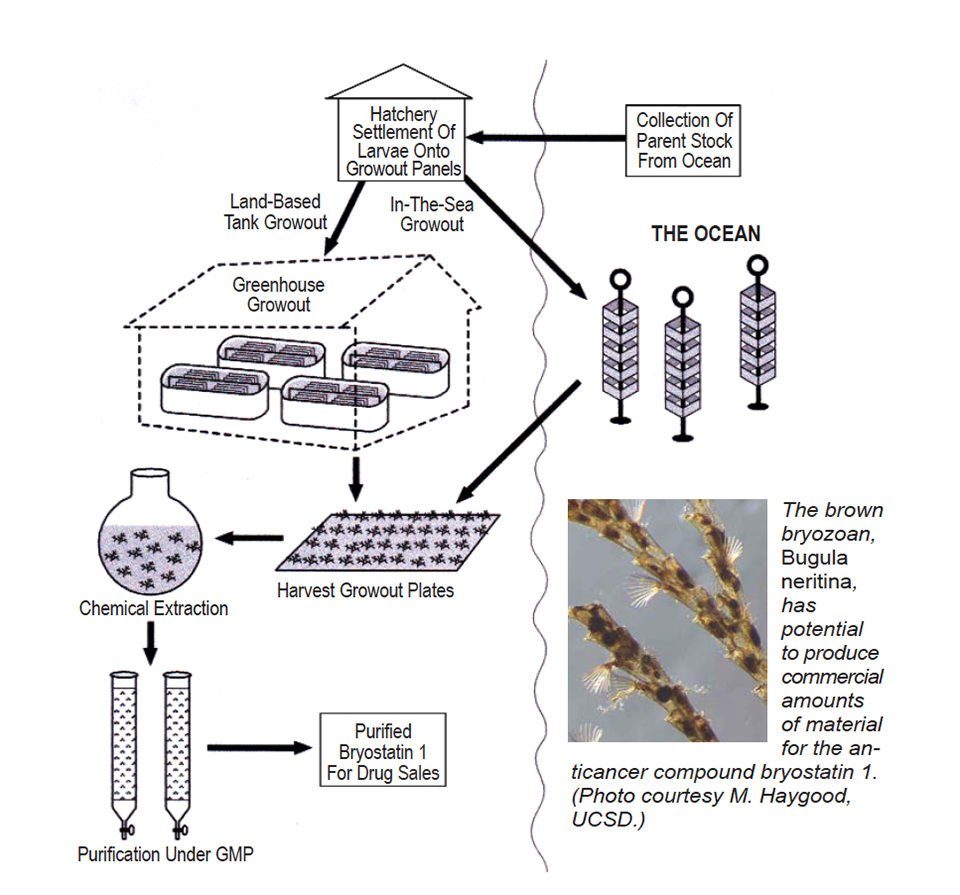
The lack of large-scale, renewable supplies of secondary metabolites derived from marine invertebrates has limited the development of new drugs from marine natural products. However, aquaculture could play a role in delivering such supplies on a commercial scale.
Bryostatin 1 from bugula neritina
Bryostatin 1, the first of a family of 20 known polyketide macrolide compounds, was discovered in 1968 from populations of Bugula neritina in waters of the northern Gulf of California by Professor G. R. Pettit of the Cancer Research Institute at Arizona State University. By the early 1990s, the highly promising marine compound had entered human clinical trials using drug supplies extracted from wild-collected biomass. Although wild collections served for clinical supplies, the quantities required for commercial drug sales (50,000 to 100,000 kilograms per biomass per year) are beyond the capacity of the natural environment to deliver in a sustainable manner.
Given that chemical synthesis would not be economical, and a fermentable source had not been discovered, aquaculture was suggested as a possible technology for providing commercial quantities of the compound. Fortunately for the culturists, B. neritina was and is the most studied marine invertebrate know to man – hundreds of scientific papers on the natural history, ecology, and reproductive biology of the organism have been published since 1758.
Feasibility studies
Aquaculture feasibility studies benefited greatly from the wealth of published information, which set the biological bases for aquaculture protocols. The scheme began with the collection of gravid broodstock colonies from near-shore rock reefs and returning them in buckets to the shore-based brood tanks. Triggered by sunlight, non-feeding ciliated larvae of 100- to 150-µ diameter were shed from ripe ovicells into the tanks at the rate of 5,000 to 7,000 larvae per kilogram broodstock. The phototrophic larvae made their way to the water surface, where they were carried over a weir and into a nested series of Nytex screens.
The collected larvae were introduced into a tall, translucent polypropylene tank with preconditioned, perforated PVC settling plates. By virtue of the geometry of the settling tank and a rectangular array of air bars set up on the tank bottom, larvae were carried by cellular water currents into the shaded spaces between the stack of plates. There they were attracted one each into the small perforations, driven by their biological imperative to find hard substratum and a quiet sanctuary for settlement and metamorphosis before their energy stores were exhausted.
In earlier studies with nonperforated unglazed tiles, hundreds of larvae settled on top of each other to form great masses of larvae in one spot. Uniform settlement was therefore indicated in the method to exclude conspecific larvae from settling onto the bare areas of the plates once they were put into the ocean. In practice, 10,000 to 30,000 larvae were required for each 0.6-m2 plate to produce a robust harvest.
Each settled larvae metamorphosed into a first “ancestrula” zooid equipped to feed by capturing plankton with a circlet of tentacles arranged around its mouth. Cultured phytoplankton of a number of strains plus artificial components were added to the tanks as a diet. With new stores of food energy, the first zooid budded asexually to form the second, then it the third, and so forth to build a new clonal colony.
The fully settled plates with the new, fragile colonies attached then were moved from the settling tank to a 4,500-liter “D”-end fiberglass raceway tank for a four- to six-week pregrowing period. At six weeks, a “fuzz” of new colonies covered most of the surfaces of the plates on both sides. From the pregrowing tanks, the plates were kept moist in racks and transferred to La Jolla Bay to be bolted onto a 7-m-long engineered steel grow-out structure anchored midway in the water column in 40 m of water.
The lab-started colonies grew well on the rich natural plankton and healthy conditions of the open sea, until, at four to five months, densely covered plates were retrieved by divers and brought to shore for harvesting of the rich mats of cultured B. neritina. Densities attained from two in-sea culture trials of 4.5 and 22 months eclipsed expectations with 2.8 and 6.0 kilogram per square meter of culture plate surface area, respectively. The bryostatin 1 content for both trials was within natural ranges, so the aquaculture process was deemed successful.
Economic analysis
An economic analysis for an in-sea system scaled to meet a projected commercial need of 100 g bryostatin 1 per year yielded a production cost in the range of U.S. $50,000 to $60,000 per grams of drug-equivalent biomass produced with $13 million in capital costs treated separately. This was considered a reasonable cost, considering that the drug was so active when injected into cancer patients. In phase II clinical trials, for example, leukemia patients each received bryostatin 1 injections totalling only 1.5 mg over an entire 18-week course of chemotherapy.
At this level of treatment, sufficient bryostatin 1 could be extracted from B. neritina biomass cultured on 200 in-sea structures to serve the needs of all the leukemia cancer patients in the United States on a renewable basis. The construction and operation of these units and a shore-based hatchery to supply the starter stock for the grow-out units are well within the state of the art of aquaculture and modern marine operations.
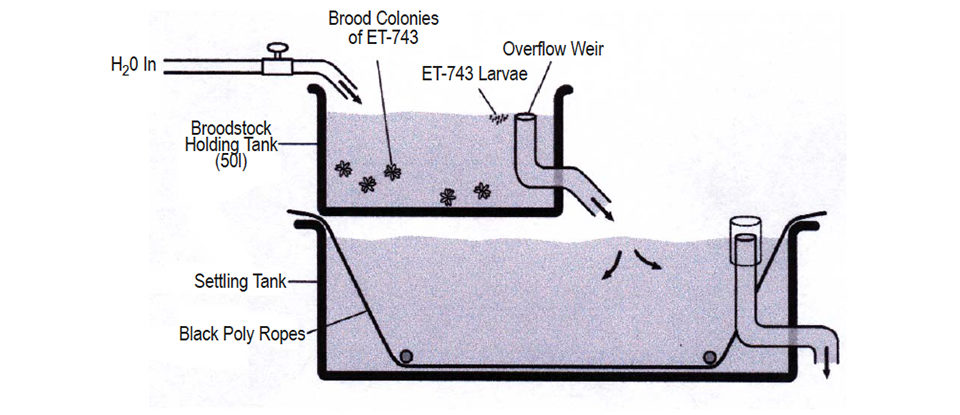
ET-743 from ecteinascidin
Ecteinascidin 743 (ET-743) is a beautiful, orange-colored colonial tunicate found throughout the Caribbean Sea as a regular colonizer on the roots of mangrove trees. A pharmaceutical company in Spain has had the compound in development since the early 1990s. It is presently in the final stages of human clinical trials for soft tissue sarcomas. The drug is expected to receive “orphan drug” marketing approvals in the European Union and United States by next year.
Aquaculture scheme
Developed at a marine laboratory in the mid-Florida Keys, the aquaculture scheme for ET-743 was essentially the same as that developed for Bugula neritina. It involved settlement of “tadpole” larvae on artificial materials (black poly ropes, in this case), followed by a pre-grow period of four to six weeks in tanks, then grow-out in the ocean in 4 to 5 meters of water on anchored PVC pipe structures.
Wet-weight harvest yields averaged 400 grams per millimeter of rope length in 60 to 90 days at sea (range: 200 to 1,200 grams per millimeter). Ecteinascidin 743 yields were equal to those from wild-collected colonies. Broodstock needs were only one-sixth of the final harvest weight per weight – a much better ratio than that required for the B. neritina process.
Another key feature of the ET-743 protocols was the fact that the cultured colonies could be harvested by stripping the ropes, leaving a basal mass of stolon material on the ropes to grow over and over again without the need for more broodstock and larval settlement. This method did not work for the Bugula process, in that once harvested by clipping, the short Bugula colonies were vulnerable to “weeding out” by settlement of other competing larvae.
Projected economics
The projected economics for a commercial-sized ET-743 aquafarm located in the mid-Florida Keys were very favorable. Projected biomass production costs over a five-year period would commence at U.S. $21.00 per kilogram fresh weight in the first year, and reduce to $14.50 per kilogram by the fifth year. Drug-equivalent biomass costs over the same period ranged $7,000 per gram ET-743 at year 1 to $2,900per gram in five years. Capital costs were treated separately.
(Editor’s Note: This article was originally published in the December 2005 print edition of the Global Aquaculture Advocate.)
Now that you've reached the end of the article ...
… please consider supporting GSA’s mission to advance responsible seafood practices through education, advocacy and third-party assurances. The Advocate aims to document the evolution of responsible seafood practices and share the expansive knowledge of our vast network of contributors.
By becoming a Global Seafood Alliance member, you’re ensuring that all of the pre-competitive work we do through member benefits, resources and events can continue. Individual membership costs just $50 a year.
Not a GSA member? Join us.
Author
-
Dominick Mendola, Ph.D.
CalBioMarine Technologies, Inc.
1001 Capri Road
Encinitas, California 92024 USA[109,111,99,46,101,110,105,114,97,109,111,105,98,108,97,99,64,97,108,111,100,110,101,109,100]
Tagged With
Related Posts
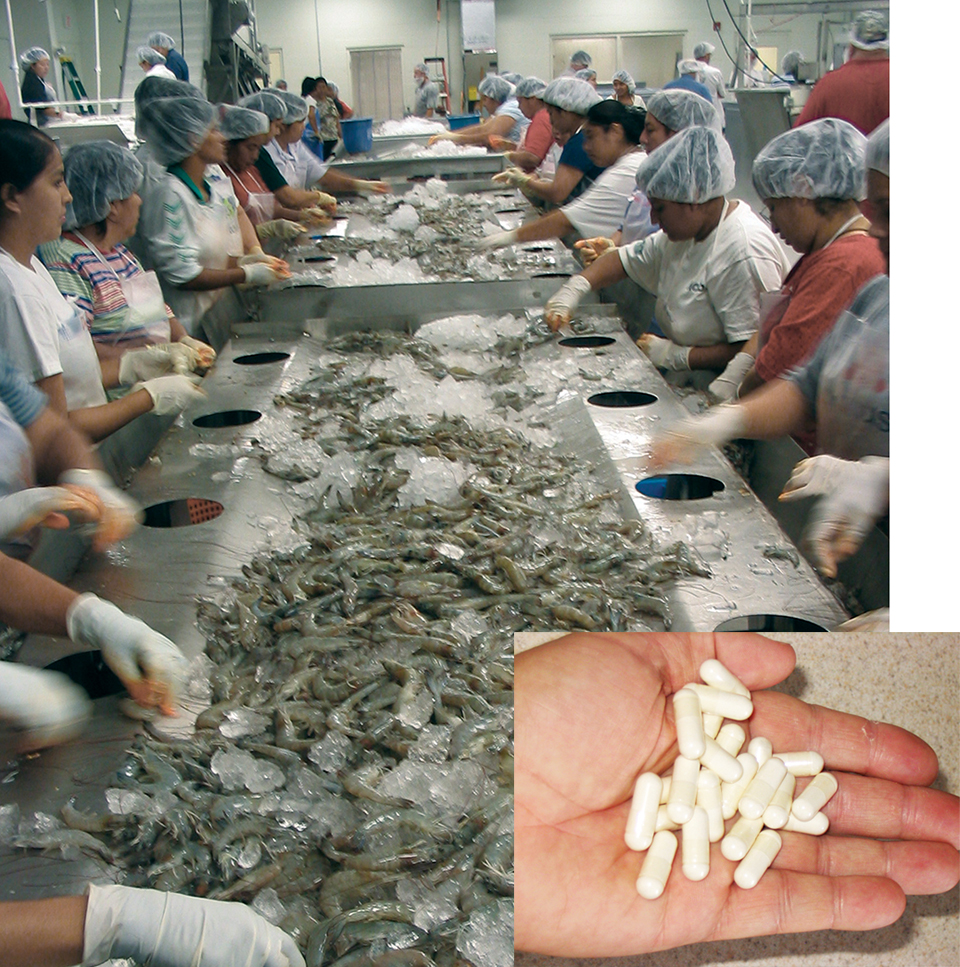
Intelligence
Shrimp processing byproducts find many uses
Shrimp processing byproducts are constructively used in shrimp or fish feeds, livestock feeds and plant fertilizer. Chitin from shrimp exoskeletons is used in bandages and varied cosmetic products.
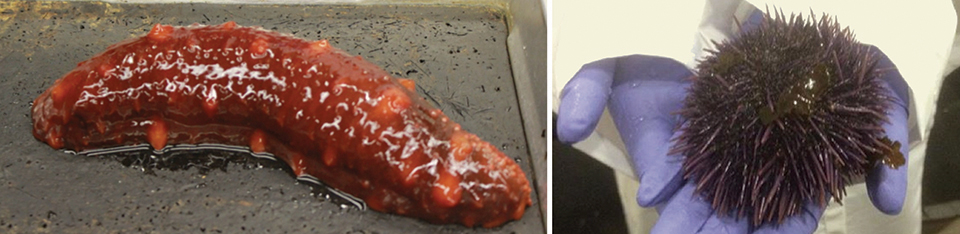
Health & Welfare
Stress susceptibility of echinoderms under aquaculture conditions
If an animal is stressed, its immune function decreases, and vulnerability to diseases increases. Since there is currently no effective treatment for disease in echinoderms, the authors examined the impacts of handling and salinity fluctuations on the immune functions of sea cucumbers and sea urchins.
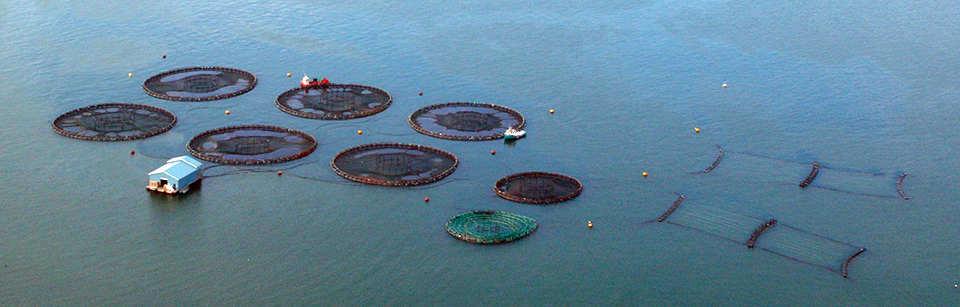
Responsibility
A look at integrated multi-trophic aquaculture
In integrated multi-trophic aquaculture, farmers combine the cultivation of fed species such as finfish or shrimp with extractive seaweeds, aquatic plants and shellfish and other invertebrates that recapture organic and inorganic particulate nutrients for their growth.
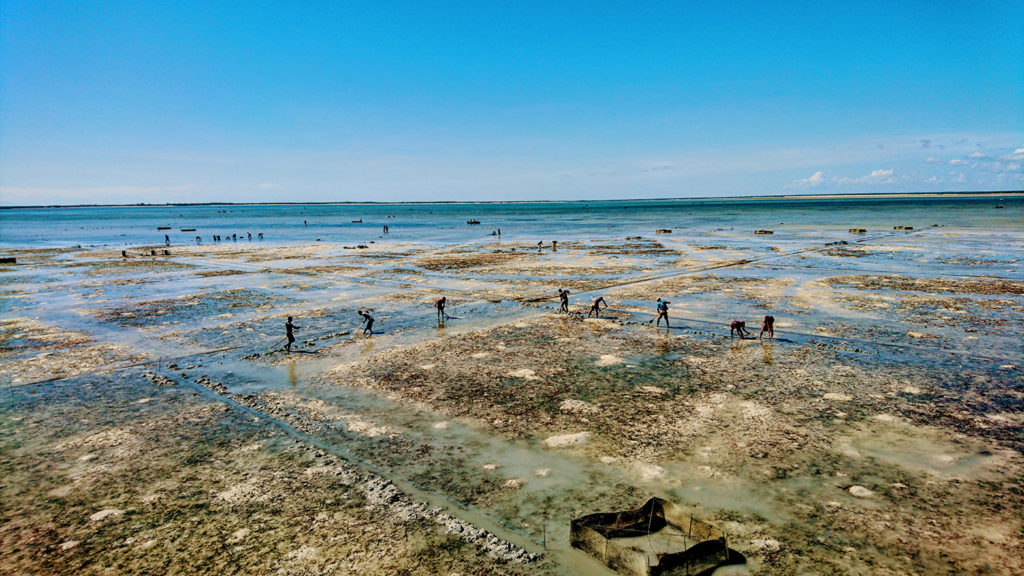
Responsibility
Sea cucumber project redefining traditional farming in Madagascar
Farming sea cucumbers – known as sea slugs in China – is changing people’s lives, giving rural workers in Madagascar a potential pathway out of poverty.